Lin Lab demonstrates new pathway for cheaper, safer, better batteries
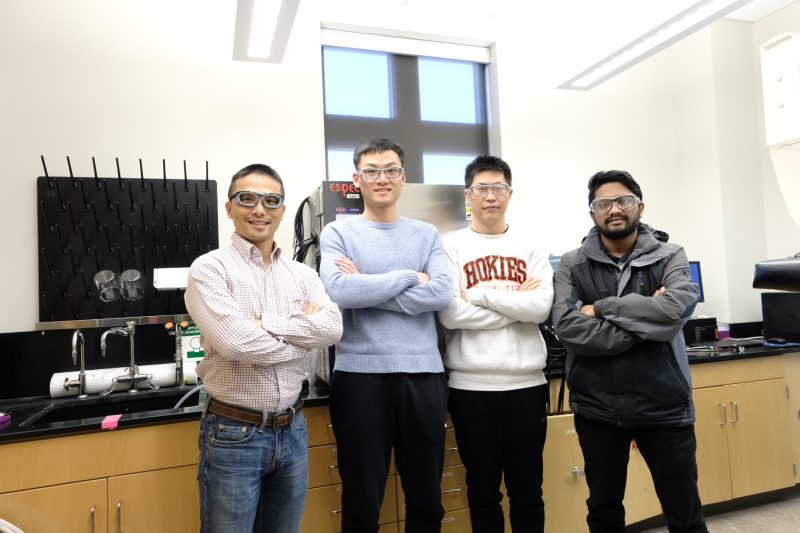
In a new journal article, Feng Lin and his team showed a promising blueprint toward cheaper, safer, and higher-performing batteries.
Lin is an assistant professor in the Department of Chemistry, and his Chemistry Ph.D. student Zhengrui Xu was the first author on the paper. The article, titled “Charge Distribution Guided by Grain Crystallographic Orientations in Polycrystalline Battery Materials” was published in Nature Communications. The study is based on an important family of high energy cathode materials, which will be implemented in the next-generation batteries for consumer electronics and electric vehicles.
“We demonstrated an easy and inexpensive way to reduce the charge heterogeneity inside the materials, which can improve the cycle life and mechanical properties of these materials,” Xu said. “It can increase the performance of these materials for our cell phones, laptops, or even electric vehicles. It sets an example for future battery development.”
Batteries consist of three main parts: two solid parts in the anode and cathode, and a liquid electrolyte that separates the two. The anode, made of graphite in commercial batteries, actually performs quite well, but the cathode is where Lin sees room for improvement. During battery usage, ions and electrons travel between the anode and cathode, and the movement of ions and electrons within the cathode is what the article investigates.
“Our research pushes the boundary of reducing the cost, and improving the safety, energy density, and lifetime of lithium ion batteries,” Lin said. “Each component in the cell has its own unique challenges, but we have to look into the battery chemistry at the system level. Solving the problems of the cathode would actually benefit the functions of other cell components.”
Previous published papers from the Lin Lab described the issues in battery charging and discharging, mostly related to uneven (heterogeneous) charging of particles in the cathode. Ideally, all cathode particles would charge at the same rate. If and when they don’t, localized areas of the cathode take on greater stress, crack, and lead to safety issues when the liquid electrolyte floods in and creates new reactions.
One of the materials used commercially in the cathode is called NMC, where N, M, C stand for nickel, manganese, and cobalt. These metal elements are compounded with lithium and oxygen to form layered oxides, the active component of the cathode. One problem with the structure of regular NMC materials is that during charging or discharging, lithium ions embedded in it must laboriously travel in a snakelike fashion to wend its way to the surface and then to the anode. Lin’s team created a new material called Rod NMC that allow ions and electrons to have direct radial outlets to the surface, allowing for a quicker release and uptake of lithium ions.
Data from the experiments showed a higher capacity with Rod NMC in addition to a higher cycle life. This means batteries with Rod NMC would have more energy and would decrease in percentage at a slower rate than batteries with regular NMC. Most importantly, the rod-like structure of Rod NMC improves the homogeneity of charging, which would lead to a safer battery.
“Our project is basic science-driven, and we have been performing this project using a range of synchrotron X-ray capabilities at various DOE national labs,” Xu said. “However, the conclusion from this fundamental study has strong implications for battery engineering and manufacturing.”
The study also involved Dr. Yijin Liu’s team at SLAC National Accelerator Laboratory. Liu is a leading expert in synchrotron sciences.
“The cutting-edge synchrotron spectro-imaging techniques played a key role in revealing the reaction mechanisms of these battery particles.” Liu said. “In addition to visualizing the fine morphological details within these particles, we also resolved the local chemical reactions that collectively govern the overall behavior of the battery cathode.”
The work received financial support from the Division of Materials Research of National Science Foundation and the Institute for Critical Technology and Applied Science at Virginia Tech. The team performed synchrotron experiments at SLAC National Accelerator Laboratory, Argonne National Laboratory, and Brookhaven National Laboratory.